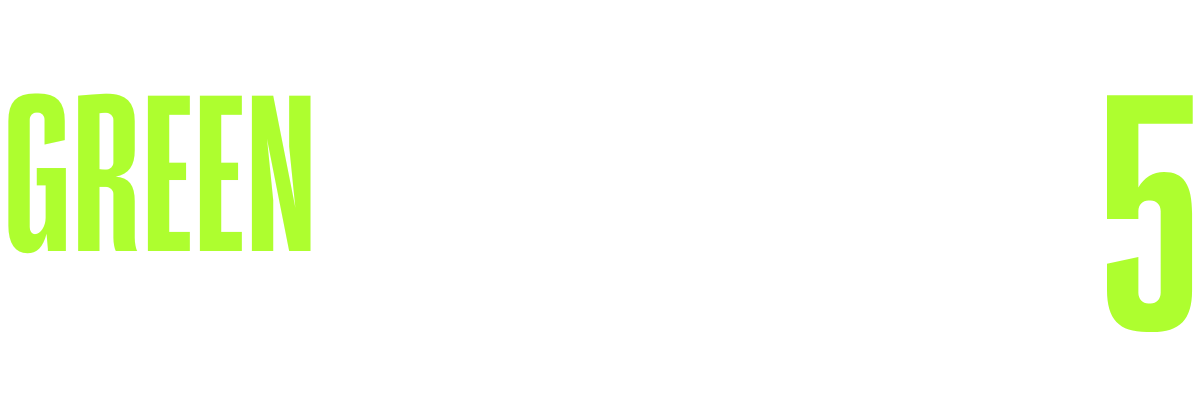
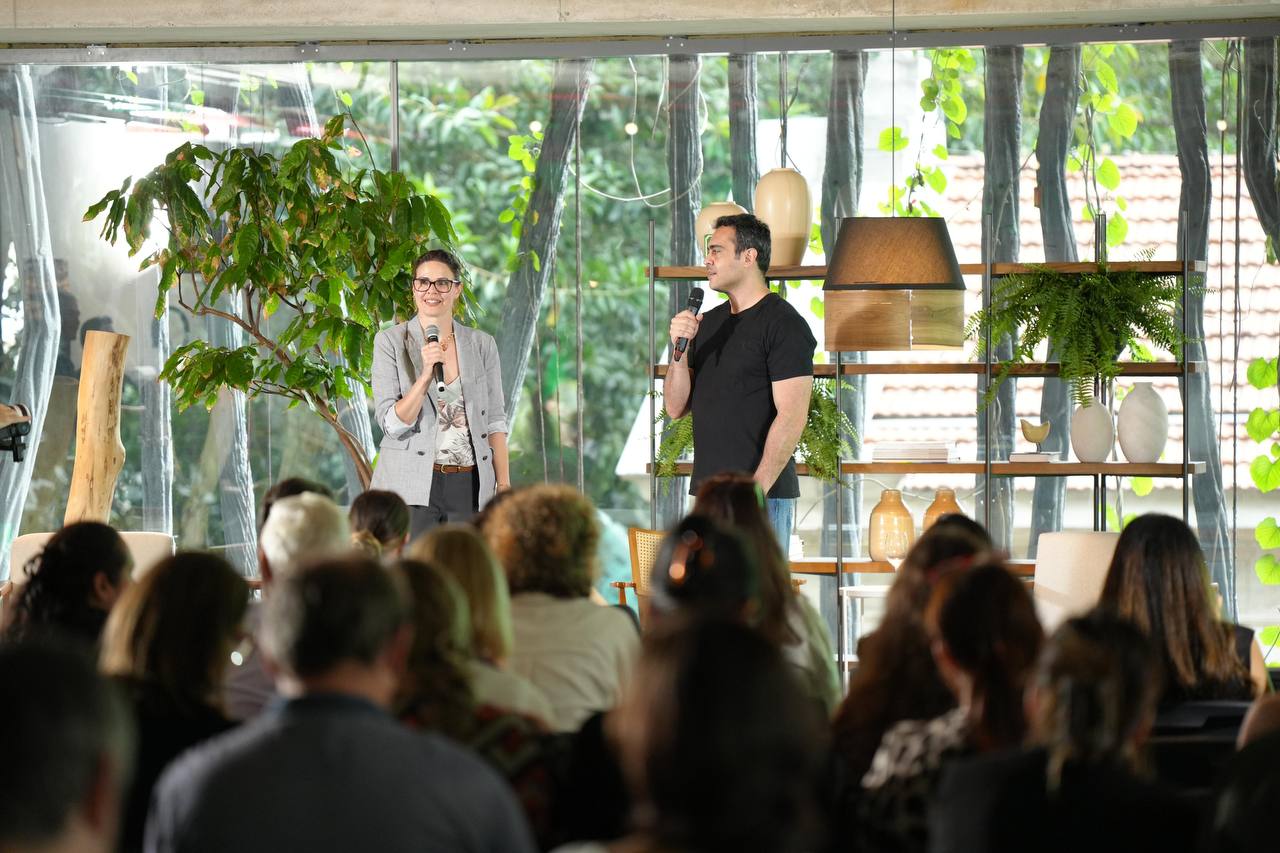
A 2-day immersion into the future of sustainable construction
In the world of sustainable architecture, understanding the concepts of embodied carbon and operational carbon is essential. These two terms play a significant role in assessing and minimizing the environmental impact of buildings throughout their life cycle. This section will provide a comprehensive introduction to embodied carbon, operational carbon, and their significance in sustainable architecture.
What is Embodied Carbon?
Embodied carbon refers to the total amount of greenhouse gas emissions associated with the production, transportation, and construction of building materials. It encompasses all the carbon emissions released during the entire life cycle of these materials, including their extraction, processing, manufacturing, and eventual disposal. Embodied carbon takes into account the carbon dioxide (CO2) emissions as well as other greenhouse gases, such as methane (CH4) and nitrous oxide (N2O).
Sources of Embodied Carbon in Construction Materials
Construction materials contribute to a significant portion of embodied carbon in buildings. Materials like cement, steel, aluminum, and plastics are known for their high carbon footprint due to the energy-intensive manufacturing processes involved in their production. Additionally, the extraction and transportation of raw materials contribute to the embodied carbon of construction materials.
Measuring and Assessing Embodied Carbon
Measuring embodied carbon is a complex task that involves evaluating the carbon emissions associated with each material used in a building project. Life cycle assessment (LCA) methodologies are commonly employed to assess the embodied carbon of different materials and construction techniques. LCA considers the cradle-to-grave perspective, analyzing the environmental impacts at each stage of a material’s life cycle.
Strategies to Reduce Embodied Carbon
Reducing embodied carbon in construction is crucial for achieving sustainable architecture goals. Various strategies can be implemented to minimize the environmental impact of building materials. These include:
- Material Selection: Opting for low-carbon or carbon-neutral materials, such as recycled or locally sourced materials, can significantly reduce embodied carbon.
- Efficient Manufacturing: Encouraging manufacturers to adopt energy-efficient processes and technologies can help reduce carbon emissions during the production of construction materials.
- Transportation Optimization: Minimizing transportation distances and utilizing sustainable transportation methods can lower the carbon footprint associated with material delivery.
- Design Optimization: Designing buildings with consideration for material efficiency, waste reduction, and ease of disassembly can contribute to reducing embodied carbon.
- Innovations in Construction Techniques: Embracing innovative construction methods, such as modular or prefabricated construction, can lead to reduced material consumption and lower embodied carbon.
Case Studies Highlighting Successful Embodied Carbon Reduction Initiatives
Real-world examples serve as inspirations for reducing embodied carbon in construction. Several noteworthy projects have successfully implemented strategies to minimize their carbon footprint. These case studies showcase the possibilities and benefits of incorporating sustainable practices:
- Project X: This project achieved a significant reduction in embodied carbon by employing recycled materials and optimizing the design for material efficiency.
- Project Y: By using locally sourced materials and implementing advanced manufacturing techniques, this project significantly lowered its embodied carbon while maintaining structural integrity.
- Project Z: Through a comprehensive life cycle assessment and innovative construction methods, this project achieved a remarkable reduction in embodied carbon and set a new benchmark for sustainable construction.
These case studies demonstrate that reducing embodied carbon is not only environmentally beneficial but also achievable through careful planning, material choices, and collaboration among stakeholders.
Operational Carbon: The Ongoing Impact
Operational carbon is a crucial aspect of sustainable architecture as it pertains to the carbon emissions resulting from the day-to-day operation and use of buildings. This section will delve into the definition of operational carbon, its sources, calculation and benchmarking methods, strategies for minimizing it, and real-world examples of effective operational carbon management.
Defining Operational Carbon
Operational carbon refers to the greenhouse gas emissions generated during the operational phase of a building’s life cycle. It includes carbon emissions resulting from energy consumption for heating, cooling, lighting, ventilation, and the use of electrical appliances within the building. Operational carbon is often associated with the burning of fossil fuels, which release carbon dioxide (CO2) and other greenhouse gases into the atmosphere.
Sources of Operational Carbon in Buildings
Buildings contribute a significant portion of global carbon emissions through their operational activities. Sources of operational carbon include:
- Energy Consumption: Heating, cooling, and lighting systems, as well as electrical appliances, consume energy, primarily derived from fossil fuels, leading to the release of operational carbon.
- HVAC Systems: Inefficient heating, ventilation, and air conditioning (HVAC) systems can result in excessive energy use and higher operational carbon emissions.
- Lighting: Traditional lighting systems, such as incandescent bulbs, are less energy-efficient compared to LED lighting, leading to increased operational carbon.
- Appliances and Equipment: Energy-consuming equipment, such as refrigerators, computers, and machinery, contribute to operational carbon emissions when powered by fossil fuel-based electricity.
Calculating and Benchmarking Operational Carbon
To assess the operational carbon of a building, energy consumption data and emission factors are used to calculate the carbon footprint. Emission factors represent the amount of CO2 or other greenhouse gases emitted per unit of energy consumed. Energy modeling software and tools enable the calculation of operational carbon based on the building’s energy consumption patterns.
Benchmarking operational carbon involves comparing the energy consumption and carbon emissions of a building to industry standards or similar buildings. Benchmarking helps identify areas for improvement and sets targets for reducing operational carbon.
Strategies for Minimizing Operational Carbon
Minimizing operational carbon is crucial for achieving sustainability goals. Strategies for reducing operational carbon include:
- Energy Efficiency: Implementing energy-efficient measures, such as insulation, efficient HVAC systems, and LED lighting, can significantly reduce energy consumption and operational carbon emissions.
- Renewable Energy Integration: Incorporating renewable energy sources, such as solar panels or wind turbines, can offset fossil fuel-based electricity consumption and reduce operational carbon.
- Smart Building Technologies: Utilizing smart technologies, such as automated energy management systems and occupancy sensors, can optimize energy use and minimize operational carbon.
- Behavior Change and Education: Promoting energy-conscious behavior among occupants through awareness campaigns and education can lead to reduced energy consumption and operational carbon emissions.
Real-World Examples of Effective Operational Carbon Management
Several real-world projects have successfully implemented strategies to minimize operational carbon. These examples highlight the effectiveness of sustainable practices in reducing carbon emissions during building operations:
- Project A: By integrating energy-efficient systems, renewable energy generation, and occupant engagement programs, this project achieved significant reductions in operational carbon emissions.
- Project B: Through the implementation of advanced building automation systems, optimized energy management, and continuous monitoring, this project achieved notable operational carbon savings.
- Project C: By utilizing passive design strategies, high-performance insulation, and efficient appliances, this project demonstrated exemplary operational carbon reduction without compromising comfort or functionality.
These examples showcase the potential for effective operational carbon management and provide valuable insights for future sustainable architecture projects.
Comparing Embodied and Operational Carbon
While embodied carbon and operational carbon both contribute to a building’s overall carbon footprint, there are key differences between the two:
- Timeframe: Embodied carbon is associated with the upfront construction and materials used, while operational carbon is ongoing and relates to the building’s energy use over its operational life.
- Scope: Embodied carbon accounts for emissions from the entire life cycle of construction materials, while operational carbon focuses solely on emissions resulting from building operations.
- Mitigation Opportunities: Strategies to reduce embodied carbon primarily involve material selection and construction practices, whereas operational carbon reduction focuses on energy efficiency measures and renewable energy integration.
- Evaluation Methods: Embodied carbon is assessed through life cycle assessments (LCAs), considering the environmental impact of materials. Operational carbon is evaluated by measuring energy consumption and associated emissions.
Understanding the Life Cycle Assessment (LCA) Approach
Life cycle assessment (LCA) is a widely used methodology to evaluate the environmental impact of buildings and materials. It considers the entire life cycle, including the extraction of raw materials, manufacturing, transportation, use, and end-of-life disposal. LCA provides a holistic perspective for assessing embodied carbon and enables informed decision-making regarding material choices and construction techniques.
Evaluating the Relative Impact of Embodied and Operational Carbon
Balancing efforts to address both embodied and operational carbon is crucial for achieving comprehensive carbon reduction in sustainable architecture. Evaluating the relative impact of embodied and operational carbon depends on factors such as building lifespan, energy efficiency, and material choices. Conducting LCAs and energy modeling helps identify areas with the most significant carbon impact and prioritize efforts accordingly.
The Role of Sustainable Architecture in Carbon Reduction
Sustainable architecture plays a vital role in carbon reduction by integrating various strategies and practices. Key considerations include:
- Carbon-Reducing Design: Architects can incorporate passive design principles, such as optimizing natural lighting and ventilation, to reduce the building’s energy demand and operational carbon emissions.
- Renewable Energy Integration: Designing buildings with renewable energy systems, such as solar panels or geothermal heat pumps, promotes clean energy generation and reduces reliance on fossil fuels.
- Material Selection: Choosing low-carbon or carbon-neutral materials with minimal embodied carbon, such as recycled or locally sourced materials, contributes to overall carbon reduction.
- Adaptability and Longevity: Designing for adaptability and longevity reduces the need for frequent renovations or demolitions, minimizing embodied carbon associated with new construction.
By adopting a holistic approach and implementing these strategies, sustainable architecture can effectively contribute to carbon reduction efforts and create a more environmentally conscious built environment.
Impacts and Benefits of Addressing Embodied and Operational Carbon
Addressing both embodied and operational carbon in sustainable architecture has a wide range of impacts and benefits that extend to the environment, occupants’ health and well-being, economics, policy and regulations, as well as future directions and challenges. This section will explore these aspects in detail.
Environmental Benefits of Reducing Embodied and Operational Carbon
Reducing embodied and operational carbon offers significant environmental benefits, including:
- Climate Change Mitigation: By minimizing carbon emissions throughout the life cycle of buildings, including construction and operation, we can contribute to mitigating climate change and reducing greenhouse gas concentrations in the atmosphere.
- Energy Conservation: Reducing operational carbon through energy-efficient measures decreases the demand for energy generation, helping conserve natural resources and decrease reliance on fossil fuels.
- Preservation of Natural Ecosystems: By minimizing embodied carbon, we can reduce the need for resource-intensive materials and construction methods, helping preserve natural ecosystems and biodiversity.
- Lower Environmental Footprint: Addressing both embodied and operational carbon contributes to a more sustainable built environment, with reduced pollution, resource depletion, and waste generation.
Health and Well-being Advantages for Occupants
Efforts to reduce embodied and operational carbon can positively impact occupants’ health and well-being, including:
- Indoor Air Quality: Sustainable architecture practices, such as selecting low-toxicity materials, improve indoor air quality, leading to better respiratory health and overall comfort for building occupants.
- Thermal Comfort: Energy-efficient buildings with optimized insulation and ventilation systems provide better thermal comfort, ensuring a healthier and more productive indoor environment.
- Natural Lighting and Views: Incorporating design elements that maximize natural light and provide access to views of nature positively affect occupants’ mental well-being, productivity, and connection to the surrounding environment.
- Noise Reduction: Implementing sustainable construction techniques and materials that minimize noise transmission can enhance occupant comfort, concentration, and overall satisfaction.
Economic Considerations and Cost Savings
Addressing embodied and operational carbon can yield economic benefits and long-term cost savings, such as:
- Energy Cost Reduction: Minimizing operational carbon through energy-efficient measures results in lower energy consumption and reduced operational costs over the building’s lifetime.
- Increased Property Value: Sustainable buildings with low carbon footprints and energy-efficient features often have higher market value and desirability.
- Operational Efficiency: Optimizing building systems and implementing renewable energy technologies can enhance operational efficiency, reducing maintenance and operational expenses.
- Future-Proofing Investments: By considering embodied and operational carbon from the outset, architects and designers can future-proof investments, anticipating stricter regulations and evolving market demands.
Policy and Regulatory Perspectives on Carbon Reduction
Policy and regulatory frameworks play a crucial role in driving carbon reduction efforts in sustainable architecture:
- Building Codes and Standards: Many countries and regions have adopted building codes and standards that incorporate carbon reduction targets, energy efficiency requirements, and sustainability criteria.
- Certification Systems: Internationally recognized certification systems, such as LEED (Leadership in Energy and Environmental Design) and BREEAM (Building Research Establishment Environmental Assessment Method), provide guidelines and criteria for achieving carbon reduction and sustainable building practices.
- Government Incentives: Governments may offer financial incentives, tax credits, or grants to promote energy-efficient and low-carbon construction projects, encouraging architects and designers to prioritize carbon reduction.
Challenges and Future Directions
While progress has been made, challenges remain in measuring and addressing embodied and operational carbon:
- Data Availability and Consistency: Access to accurate and comprehensive data on carbon emissions associated with materials and operational energy use can be limited, hindering precise carbon accounting and benchmarking.
- Technological Limitations: Current methodologies for assessing embodied and operational carbon may lack standardization and can be time-consuming and resource-intensive.
- Industry Awareness and Education: Bridging knowledge gaps and raising awareness among professionals about the significance of embodied and operational carbon is essential to drive widespread adoption of carbon reduction strategies.
To overcome these challenges, advancements in carbon accounting methodologies and tools are continuously being made, including the development of digital platforms and databases that streamline data collection, analysis, and reporting. Additionally, the integration of Building Information Modeling (BIM) technology enables more accurate assessments of embodied carbon at early design stages.
Looking ahead, future developments in sustainable architecture and carbon reduction may include:
- Material Innovations: Advancements in low-carbon and carbon-neutral materials, such as engineered timber and recycled materials, offer promising alternatives to high-emission construction materials.
- Net Zero and Positive Energy Buildings: The rise of net-zero energy buildings and positive energy buildings demonstrates the potential for buildings to generate renewable energy and contribute to the grid.
- Circular Economy Principles: Embracing circular economy principles can minimize waste and maximize the reuse, recycling, and repurposing of building materials, reducing embodied carbon associated with new construction.
- Policy and Regulatory Strengthening: Strengthening policies and regulations related to embodied and operational carbon, including stricter energy performance standards and mandatory carbon reporting, will encourage industry-wide adoption of sustainable practices.
Collaboration and industry-wide efforts are vital to accelerate progress in addressing embodied and operational carbon. Architects, engineers, designers, policymakers, and other stakeholders must collaborate, share knowledge and best practices, and work towards a common goal of achieving a sustainable, low-carbon future.
Conclusion
In conclusion, embodied and operational carbon have significant implications for sustainable architecture. By addressing both aspects, we can minimize the environmental impact of buildings, enhance occupant health and well-being, achieve cost savings, and align with evolving policy and regulatory frameworks. Architects, engineers, and designers have a crucial role to play in prioritizing carbon reduction through innovative design, material selection, energy-efficient systems, and a commitment to continuous improvement. By adopting sustainable practices and embracing the opportunities presented by carbon reduction, we can shape a built environment that is environmentally responsible, economically viable, and beneficial for present and future generations.
Call to Action for Architects, Engineers, and Designers
As professionals in the field of sustainable architecture, architects, engineers, and designers have the power to drive positive change. Here are some actions you can take to prioritize carbon reduction:
- Educate Yourself: Stay updated on the latest research, trends, and best practices related to embodied and operational carbon. Continuously expand your knowledge and expertise in sustainable architecture.
- Integrate Life Cycle Thinking: Consider the life cycle impacts of buildings and materials from inception to end-of-life. Integrate life cycle assessment (LCA) methodologies into your design and decision-making processes.
- Collaborate and Share: Engage in collaborative efforts with other professionals, industry organizations, and researchers to share experiences, knowledge, and success stories. Together, we can accelerate progress towards carbon reduction goals.
- Advocate for Change: Be an advocate for sustainable architecture and carbon reduction. Educate clients, colleagues, and stakeholders about the benefits of carbon reduction strategies and the importance of sustainable design principles.
- Stay Innovative: Embrace innovation and explore emerging technologies, materials, and practices that can further enhance carbon reduction efforts in the field of sustainable architecture.
Empowering Individuals to Access Further Resources and Education
To learn more about sustainable architecture and carbon reduction practices, there are various resources available:
- Professional Organizations: Join professional organizations related to sustainable architecture, such as the U.S. Green Building Council (USGBC), Royal Institute of British Architects (RIBA), or Green Building Councils in your respective countries. These organizations offer resources, training programs, and networking opportunities.
- Online Platforms and Courses: Explore online platforms that offer courses and educational materials on sustainable architecture, carbon reduction, and green building practices. These platforms include Coursera, edX, and the American Institute of Architects (AIA) online learning platform.
- Research and Publications: Stay informed by reading research papers, reports, and publications from reputable sources such as academic journals, industry publications, and sustainability-focused organizations like the World Green Building Council (WGBC) and the Intergovernmental Panel on Climate Change (IPCC).
By empowering individuals with the knowledge and resources needed to embrace sustainable architecture and carbon reduction, we can collectively create a built environment that is resilient, efficient, and environmentally responsible.
FAQs
- What is the difference between embodied carbon and operational carbon? Embodied carbon refers to the emissions associated with the extraction, manufacturing, transportation, and construction of building materials. Operational carbon, on the other hand, refers to the carbon emissions resulting from the energy consumption and operation of buildings.
- How can architects and designers reduce embodied carbon in construction projects? Architects and designers can reduce embodied carbon by prioritizing low-carbon materials, using locally sourced and recycled materials, optimizing building designs for material efficiency, and adopting sustainable construction methods.
- What are some strategies for minimizing operational carbon in buildings? Strategies for minimizing operational carbon include implementing energy-efficient systems and technologies, optimizing building insulation and air sealing, utilizing renewable energy sources, promoting occupant behavior change, and employing smart building management systems.
- Are there any international standards or certifications related to embodied and operational carbon? Yes, there are international standards and certifications that address embodied and operational carbon. Examples include LEED (Leadership in Energy and Environmental Design), BREEAM (Building Research Establishment Environmental Assessment Method), and the Living Building Challenge, which promote sustainable building practices and carbon reduction.
- How can I learn more about sustainable architecture and carbon reduction practices? You can learn more about sustainable architecture and carbon reduction practices by attending workshops, conferences, and webinars; reading books and research papers on the subject; and accessing online resources and educational platforms provided by reputable organizations in the field of sustainable architecture and green building practices.
Did you like the content? So go to our youtube channel and watch our videos about the animated architecture glossary too!
Click on the sentence below:
Embodied and Operational Carbon For Green Buildings – Sustainable Architecture Animated Glossary.